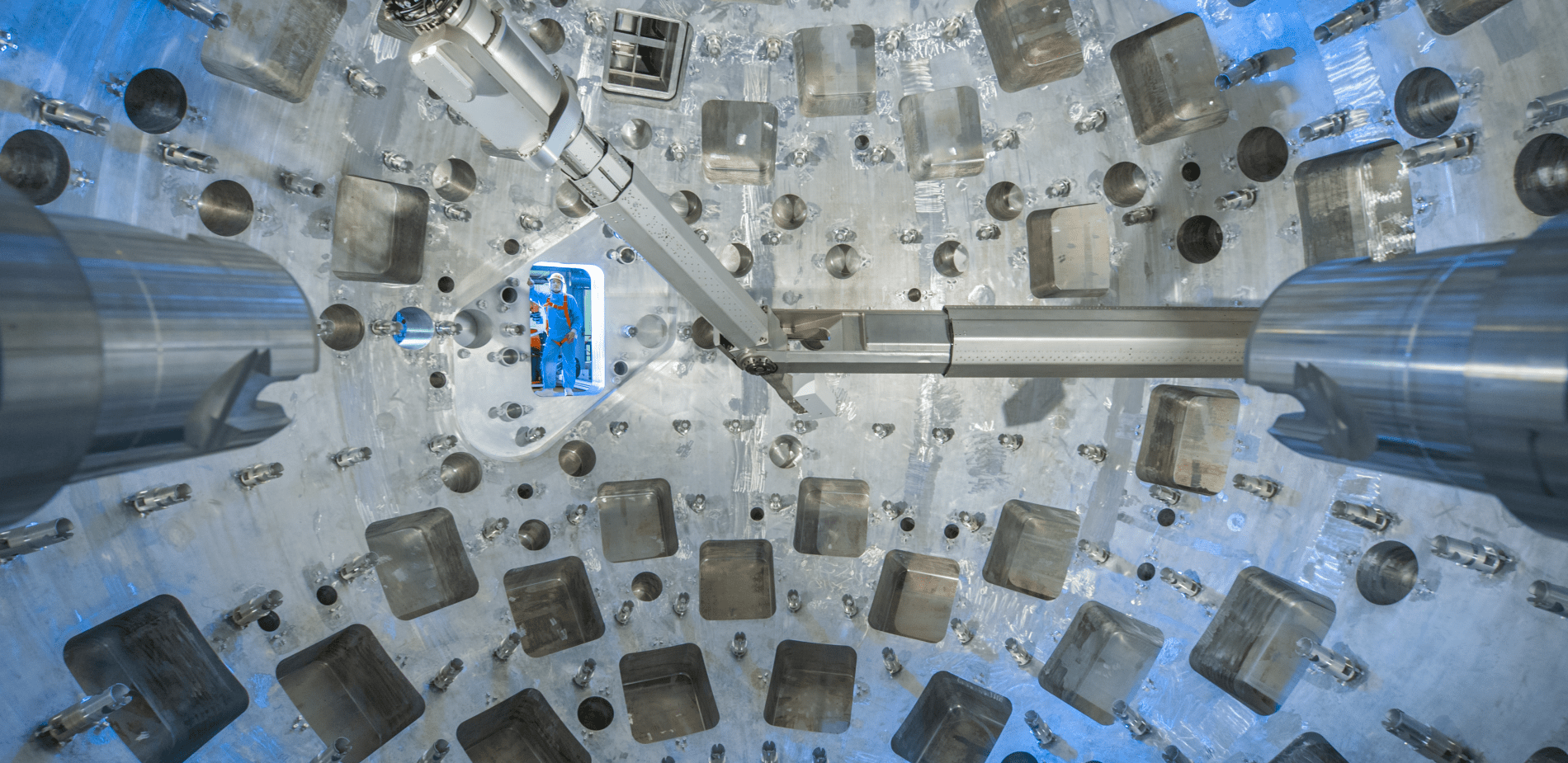
Nuclear fusion, a mature phase?
After decades of effort, nuclear fusion is now providing its first proof of concept. But is the availability of this energy source, reputed to be clean and inexhaustible, really just around the corner?
Report by Vahé Ter Minassian - Published on
The fusion race has been relaunched
On 30 July 2023, scientists at the Lawrence Livermore National Laboratory (National Ignition Facility, NIF) in California repeated the feat of using nuclear fusion to create a plasma that emits more heat than it receives. Eight months earlier, on 5 December 2022, the same team had managed to achieve this milestone for the first time in the history of physics. This feat was significant enough for the announcement to be made by the US Secretary of Energy herself, Jennifer M. Granholm, at an emergency press conference: the attention paid to the NIF results was equal to the feat. There is no doubt that these experiments will go down in the history of science. At the very least, they will be remembered as milestones in the long process initiated with a view to one day mastering a phenomenon at work in stellar cores, and also in H-type thermonuclear weapons: fusion. This nuclear reaction – as distinct from nuclear fission in today’s power stations – has been the focus of physicists’ attention for decades. The tremendous amount of heat it generates holds out the promise of a new, inexhaustible energy source that is clean and does not emit greenhouse gases. So much so that, as the announced deadlines were pushed back, it became seen as a kind of myth in physics. Perhaps wrongly, because progress is being made. Science is providing proof. A new generation of instruments is coming into operation. And industry is beginning to show an interest in academic research. Has fusion entered a mature phase?
Energy from the Sun in the lab?
Can we reproduce the phenomena that cause the Sun to heat up? Not exactly. Our star draws most of its energy from its enormous reserves of hydrogen. But the chain reactions, consisting of a succession of fusions between atomic nuclei, are not very efficient and, in any case, cannot be reproduced on Earth. Among the solutions explored in the laboratory, physicists favoured a deuterium-tritium mix very early on. The fusion of these two heavy hydrogen isotopes results in the release of a helium-4 nucleus and a neutron. And it generates a lot of heat!
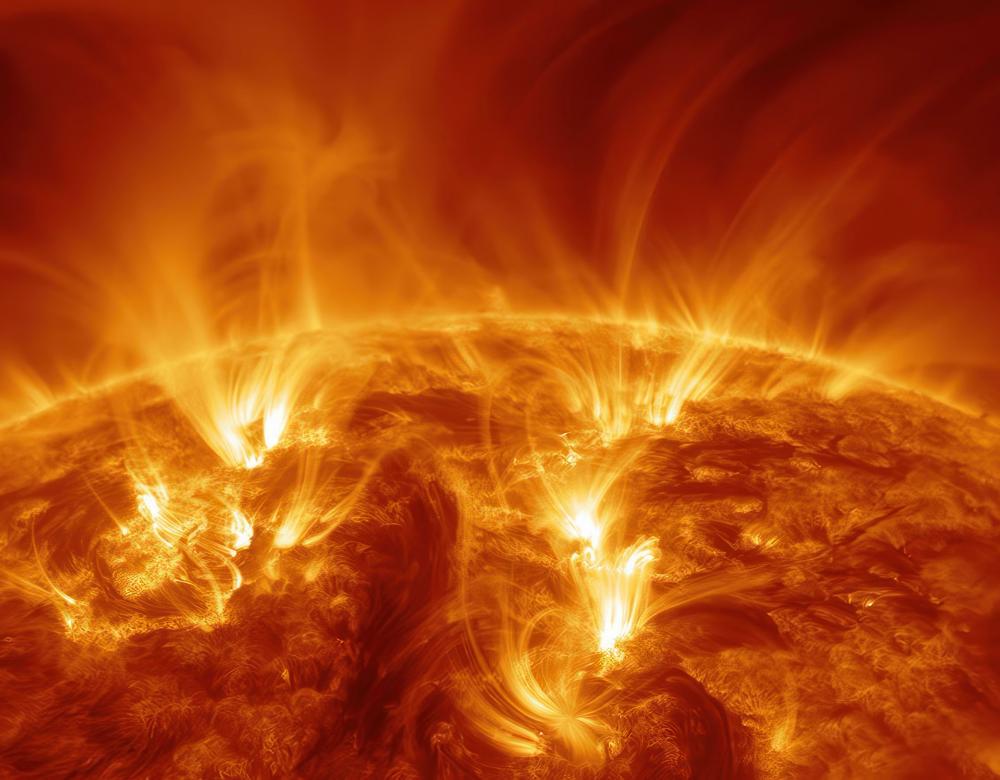
A century of history, two technological paths
In 1932, Australian physicist Marcus Oliphant caused two atomic nuclei to fuse through collision in a particle accelerator. But the war soon consigned this type of research to military secrecy, and it was not resumed until some twenty years later. After the Conference on the Peaceful Uses of Atomic Energy organised by the United Nations in 1958, the Soviets and Americans agreed to cooperate on research into fusion power generation. Two concepts were identified for controlling thermonuclear reactions. In order for deuterium and tritium gases to generate a surplus of energy, they have to be heated. This means producing a plasma at 150 million degrees – a temperature ten times higher than that of the Sun’s core – that is sufficiently dense and durable to allow atomic nuclei to meet. The second objective is to ensure that the resulting fusions contribute to maintaining the reactions by the heat they impart to the rest of the plasma. Since no chamber is capable of withstanding such constraints, an initial idea was to contain the plasma using magnetic fields. This is the “magnetic confinement” approach that led to the development of “tokamaks” by the Soviets in the 1950s, and that has been adopted by the international ITER project. In 1962, researchers at the Lawrence Livermore National Laboratory (NIF) in the United States proposed using lasers to concentrate the deuterium-tritium mix in a tiny volume in order to “ignite” the fusion reactions. This is the “inertial confinement” approach.

First ignition, an essential milestone
One of the current challenges in fusion is to obtain more energy than the amount supplied to initiate the reaction: this is “ignition”. This step was taken for the first time on 5 December 2022 in California by the NIF (National Ignition Facility) teams. A set of lasers supplied some 2.05 megajoules (MJ) to a deuterium-tritium capsule, which released 3.15 MJ after consuming around 8% of the fuel. But to do this, the NIF’s 192 lasers used several hundred times more electricity! The feat was repeated at the end of July 2023 by the same team, with improved efficiency since the energy produced was estimated at more than 3.5 MJ: enough to power an iron for an hour!
Inertial confinement at the NIF and LMJ
A number of processes are aimed at achieving “fusion by inertial confinement”. The best known, developed both at the NIF in California in the United States and at the CEA’s Laser Megajoule (LMJ) near Bordeaux in France, uses gigantic lasers occupying buildings several hundred metres long. On 5 December 2022, NIF researchers directed the beams of 192 of these optical instruments towards the inside of an experimental chamber ten metres in diameter, with a one-centimetre hollow cylinder at its centre. By heating the inner walls of this cavity, the team created a “bath” of X-rays that vaporised the surface of a deuterium-tritium capsule the size of a peppercorn. This suddenly expanded, creating a shock wave that travelled at 400 km/s towards the central part of the fuel. Compressed and heated in this way, the inner layers of the capsule fused. Although also used for civilian research in fields such as geophysics and astrophysics, the NIF and LMJ have primarily military objectives. Designed as part of nuclear deterrence programmes, these gigantic facilities are responsible for carrying out experiments to feed the “simulation codes” that describe how thermonuclear weapons work, without having to resort to full-scale tests that are far more problematic. Although they are not intended to develop energy production, they do enable new knowledge to be acquired in the understanding and control of fusion.
Laser Mégajoule : building momentum
Commissioned in 2014 in Barb, not far from Bordeaux, the CEA’s Laser Mégajoule (LMJ) continues to build momentum, with 88 lasers in operation this year, plus an 89th laser, the PETAL beam, dedicated to academic research. By 2025, 176 beams will equip this facility, which should be fully operational by 2027. The LMJ will not be looking to break records straight away. In fact, the first fusion tests in its programme do not involve the use of a deuterium-tritium mix capable of reaching the ignition threshold. Above all, they will help to improve the simulation models used by military research as part of the nuclear deterrent.
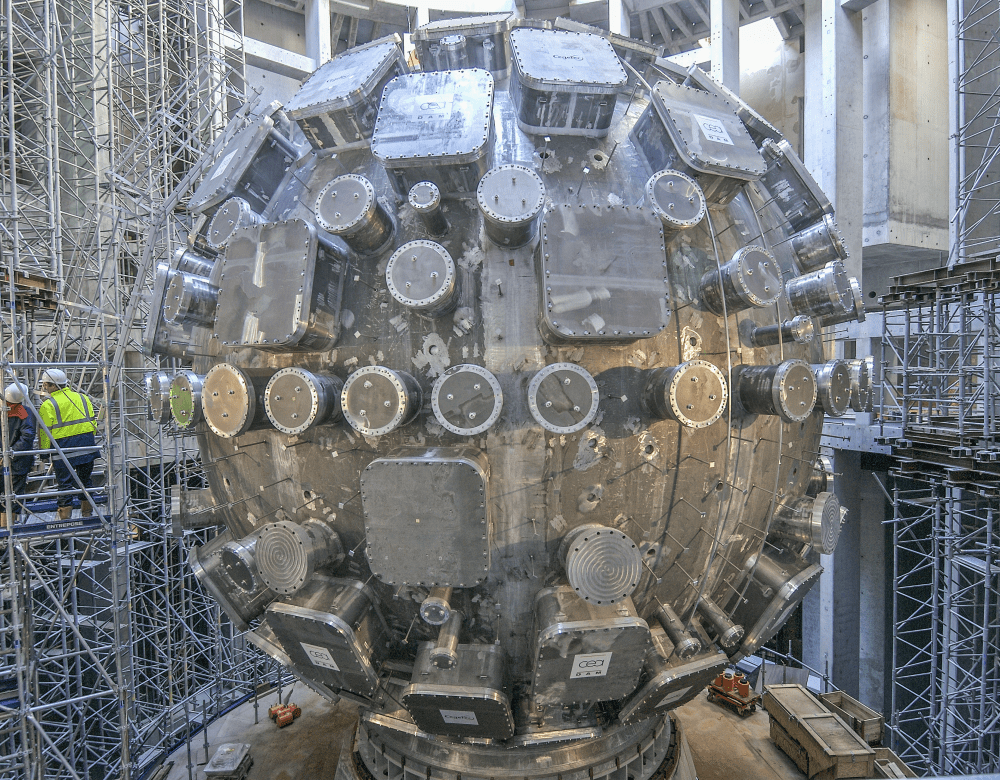
Fusion : two Technological avenues
Two main technological avenues are being explored to control fusion between two atomic nuclei: inertial confinement and magnetic confinement (not available in English).
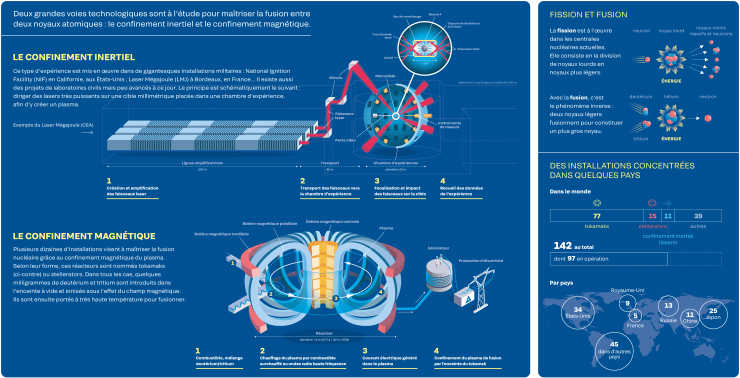
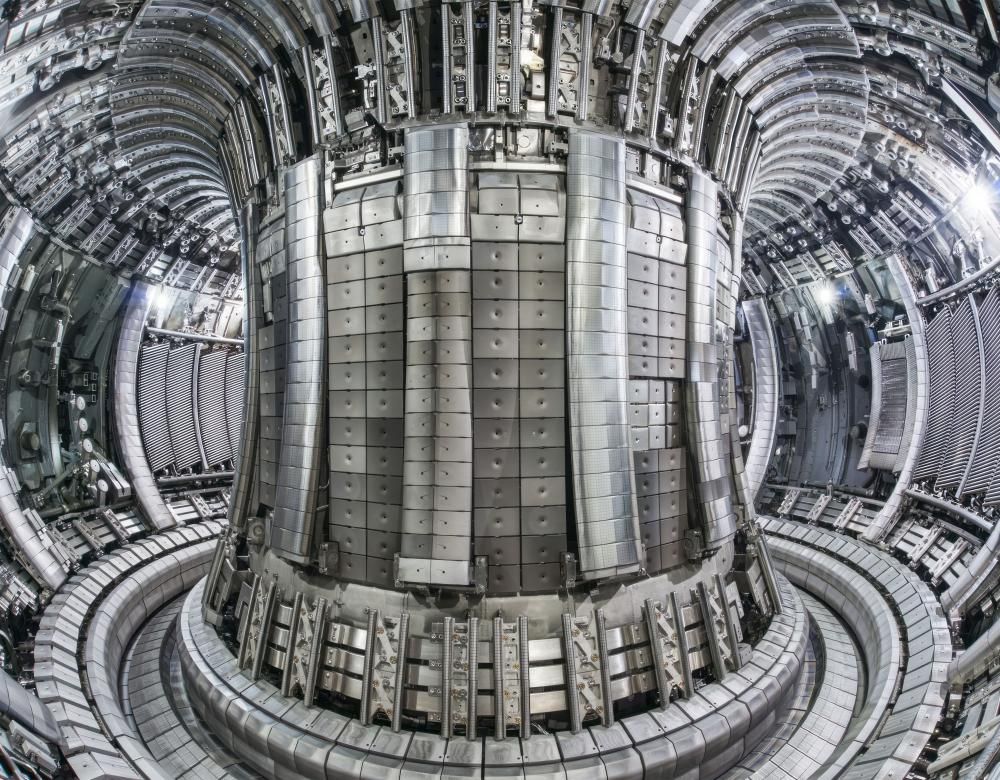
Fusion record at JET, good news for the ITER?
On 21 December 2021, the largest of all the tokamaks in operation, the JET (Joint European Torus), installed in Abingdon (UK), broke its own world record for fusion energy. It created a deuterium-tritium plasma that produced 59 megajoules of energy (equivalent to burning one kilogramme of petrol) for five seconds. According to physicists, the test demonstrates that the technological choices made for the construction of the future international ITER facility in Cadarache, France, are the right ones.
ITER, the magnetic confinement option
On 21 November 2006, China, South Korea, the United States, India, Japan, Russia and the European Union signed the final agreement for the construction of ITER. Proposed twenty years earlier by Soviet leader Mikhail Gorbachev, work on this civil nuclear research reactor can now begin near the Cadarache site in the Bouches-du-Rhône region of France. The aim is to demonstrate that fusion is a viable energy source for large-scale power generation. The infrastructure, covering an area of 42 hectares, is 85% complete. The assembly of the site’s centrepiece – a gigantic experimental tokamak – began in May 2020. This extraordinary reactor will use 10,000 tonnes of superconducting magnets to generate the magnetic fields needed to maintain 840 m3 of molten plasma in a vacuum chamber with a radius of 6 metres. The challenges are colossal. It is a human challenge, since it involves assembling the largest machine of its kind from components supplied by 35 countries, and getting researchers from all these countries to collaborate. It is also a scientific challenge, because ITER has to prove that a tokamak is capable of delivering 500 mW of thermonuclear power for 400 seconds, and therefore that a plasma in fusion can produce ten times more energy than it receives. ITER should also help physicists to control the magnetohydrodynamic instabilities that can occur during combustion, which could damage the reactor. Finally, the installation will explore the possibility of producing, within the vacuum vessel itself, the tritium used for fusion reactions. Unlike deuterium, this radionuclide is rare and expensive.
On the ITER site, assembly and dismantling
Located in Saint-Paul-lez-Durance, in the south of France, ITER has been one of Europe’s largest construction sites since 2007, employing more than 2,500 people from some 500 companies, 80% of them French. The 350,000 m3 Tokamak Building is currently being used to assemble the device, which comprises 6 giant annular magnets measuring 17 and 24 metres in diameter. But the project has not been without its problems. In late 2022, the discovery of welding defects led to the postponement of the first plasma to 2030. In 2023, 3 tokamak modules have been found to be defective and had to be disassembled, which could add to the delay and cost of the programme.

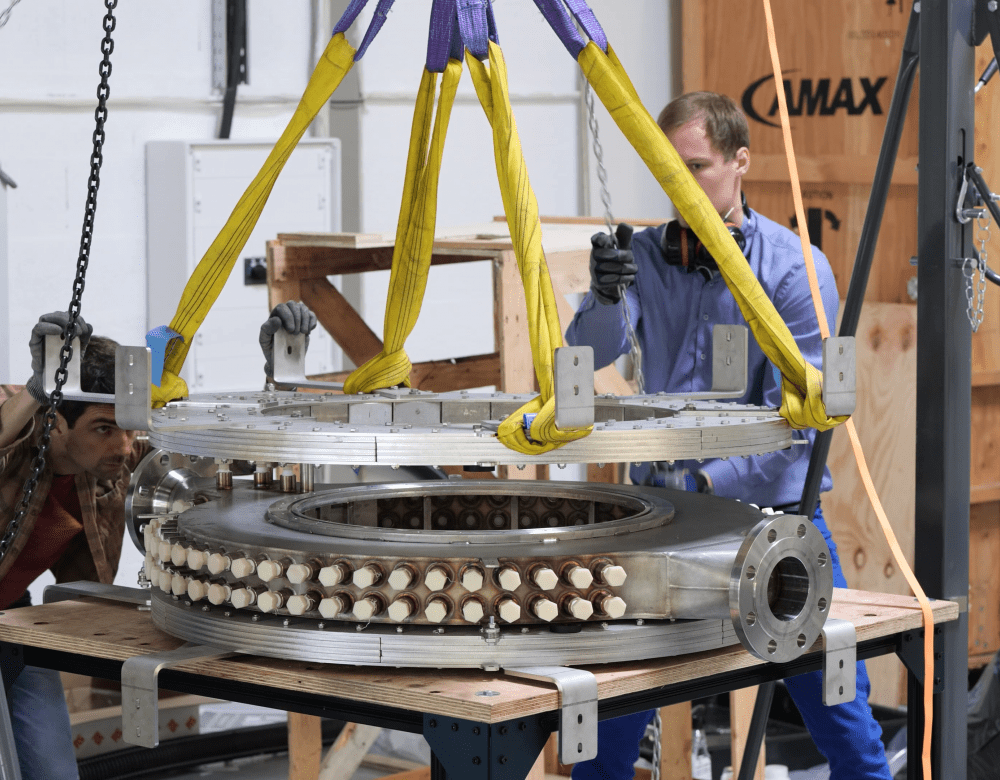
United States, Europe: start-ups get involved
An unlimited form of energy, reputed to be clean and waste-free, is sure to attract investors. Long confined within the walls of public laboratories, research into nuclear fusion seems to have reached a sufficient degree of maturity to interest industry. Several privately-funded projects have been launched in the United States and Europe, leading to the creation of around thirty start-ups. In France, Renaissance Fusion offers superconducting magnet technologies and liquid metal divertors to confine stellarator plasmas (a variant of tokamaks).
Should you bet on fusion power?
With an experiment on 5 December 2022, physicists demonstrated the possibility of generating more energy from a fusion plasma than is needed to maintain the reaction. And not in an uncontrolled way like in 1952, with the explosion of Ivy Mike, the very first H-bomb in history, but in a controlled way. Now this scientific hurdle has been cleared, has this opened the way for the industrial exploitation of a new energy source? The connection of the first thermonuclear reactors to the electricity grid over the next decade, as promised by certain companies, seems unlikely. There are still many challenges to overcome, starting with energy efficiency: to exceed the ignition threshold, the NIF lasers used hundreds of times more electricity than they helped to produce. Another obstacle is the availability of fuel: while deuterium is relatively easy to obtain, the same cannot be said for tritium, a nuclear waste product whose global stock of 25 kilograms is well below the needs of an industrial sector. Another difficulty is the impact on the environment. Fusion is intended to be a clean source of energy, but it uses neutrons to heat a heat transfer fluid. These particles make certain materials radioactive. Finally, we need to choose the technology – tokamak or stellarator, another plasma confinement device – and the fuels. The first answers will be provided by the commissioning of the future DEMO demonstration reactors connected to the grid, on the ITER site. In other words, not before 2035, for commercial operation in 2040 at best, and widespread use by the end of the century. When it comes to fusion, it's best to be patient.
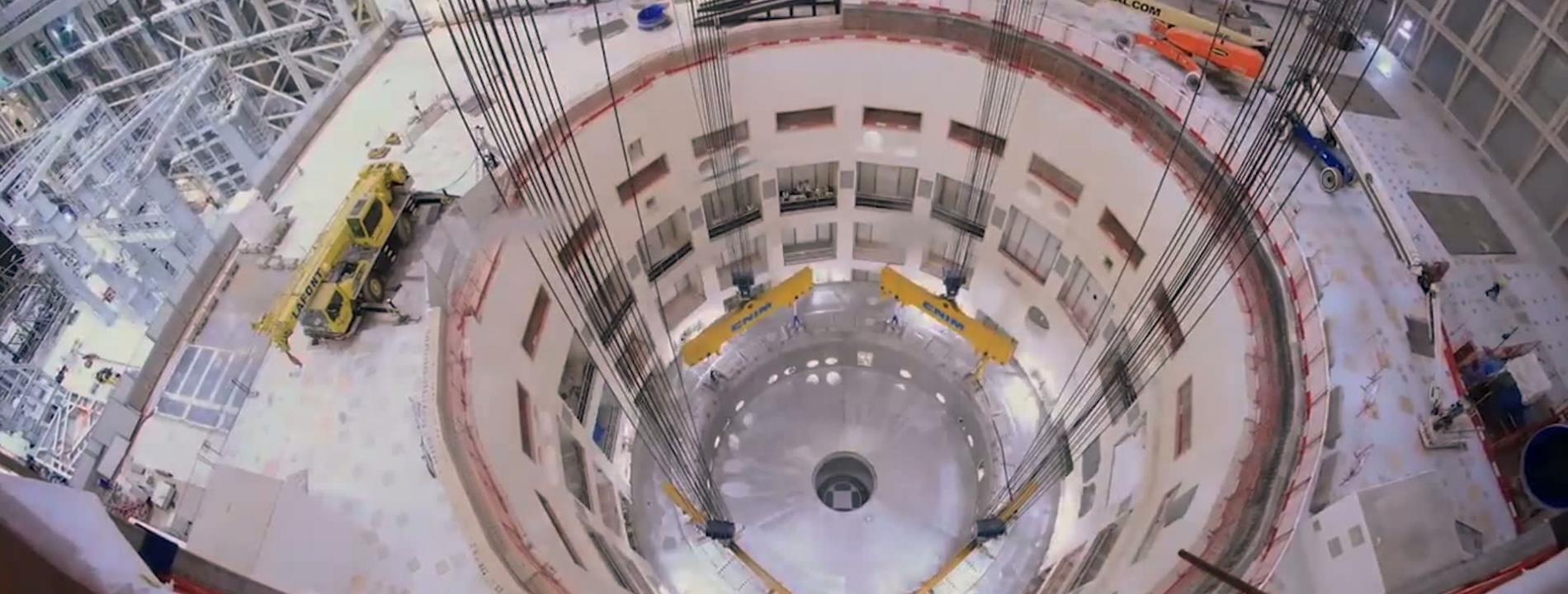