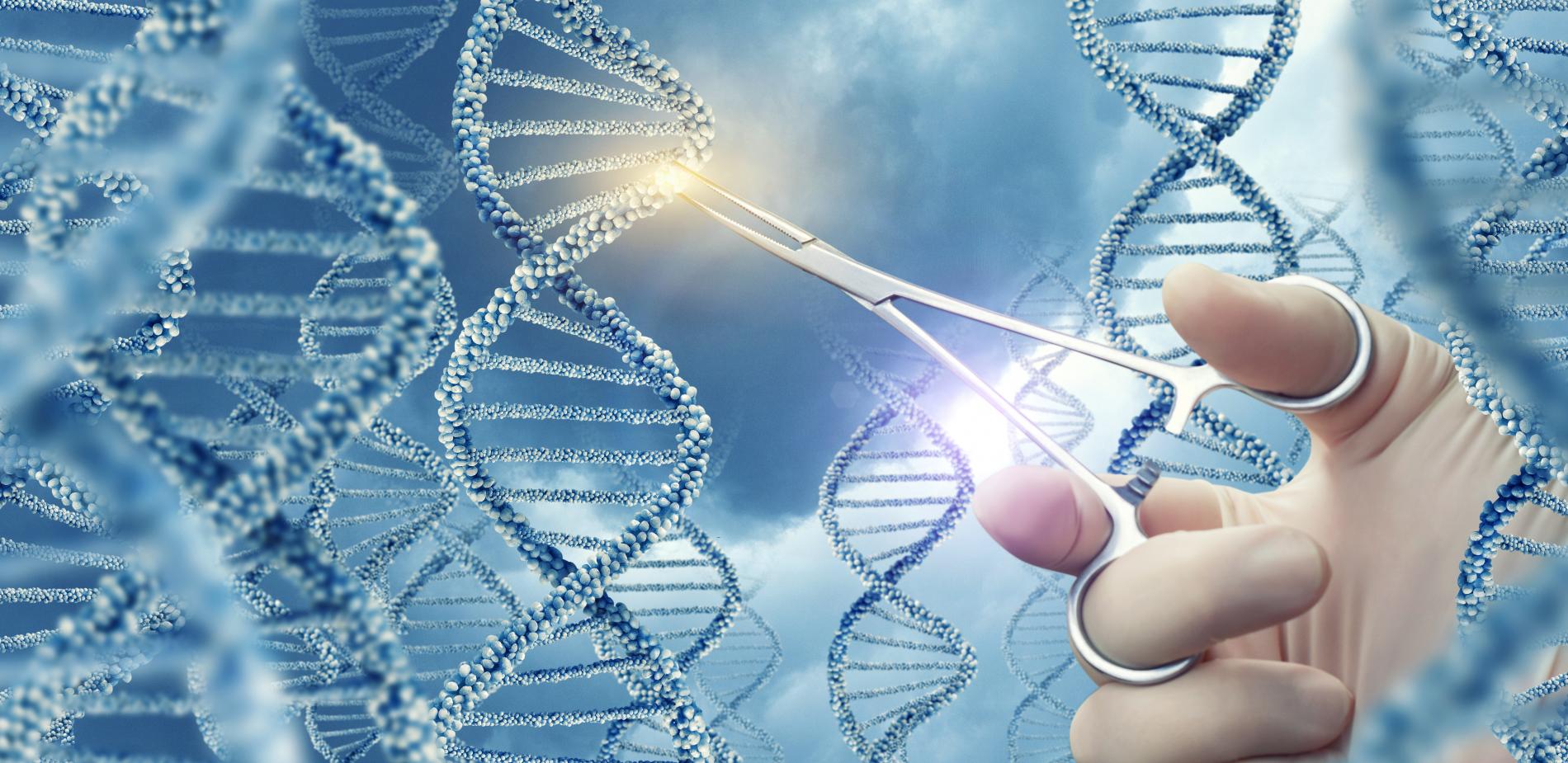
Crispr-Cas9: combining hope and caution
Ten years after its discovery, Crispr-Cas9 is in daily use in laboratories. From medical applications and plant adaptations to the “de-extinction” of species, this genome-editing tool offers breathtaking prospects.
Report by Lise Barnéoud - Published on
“Crispr madness” is evident everywhere
Hundreds of thousands of laboratories are using it all over the world. Some fifty clinical trials are underway, with 200 people already treated. Agricultural applications are available on the North American and Japanese markets. Rarely has a discovery in fundamental science led to such radical changes in such a short space of time. Fifteen years ago, what was known as Crispr (for Clustered Regularly Interspaced Short Palindromic Repeats) was a mere scientific curiosity: a defence mechanism used by bacteria to detect and cut the DNA of viruses that infect them. But in 2012, French microbiologist and geneticist Emmanuelle Charpentier along with American Jennifer Doudna demonstrated that this mechanism could be programmed to target any section of DNA and cut it. The result was a revolutionary tool for genetic targeting and genome editing, described in their landmark article published in the journal Science in June 2012. By 2013, the same journal was talking about “Crispr madness”: dozens of laboratories were using the tool to delete, add or activate genes in the cells of bacteria, mice, rats, fish, plants and human beings. Ten years on, the range of uses for Crispr-Cas9 has expanded: fine genome editing, but also editing RNAs produced by genome transcription, and editing epigenomes – the markers that influence how our genes are read. Crispr and its derivatives have become indispensable to research. The first “Crispr therapy” is expected to be available before the end of 2023. In short, things are moving very fast. Too fast, perhaps. Because beyond the uncertainties about its risks, there are ethical and societal issues to consider.
The first human face of Crispr-Cas9
Victoria Gray was one of the first patients to be treated with Crispr, in 2019. Four years later, in 2023, when she told her story at the third International Human Genome Editing Summit, the emotion was palpable. The tool has made it possible to cut out a faulty gene responsible for the most common genetic disease: sickle cell anaemia. And that’s not all. The follow-up data from the 83 people included in this clinical trial is very encouraging. By the end of 2023, this trial could lead to the first international regulatory approval of Crispr therapy. But its cost, in excess of one million euros, will drastically limit access to it.
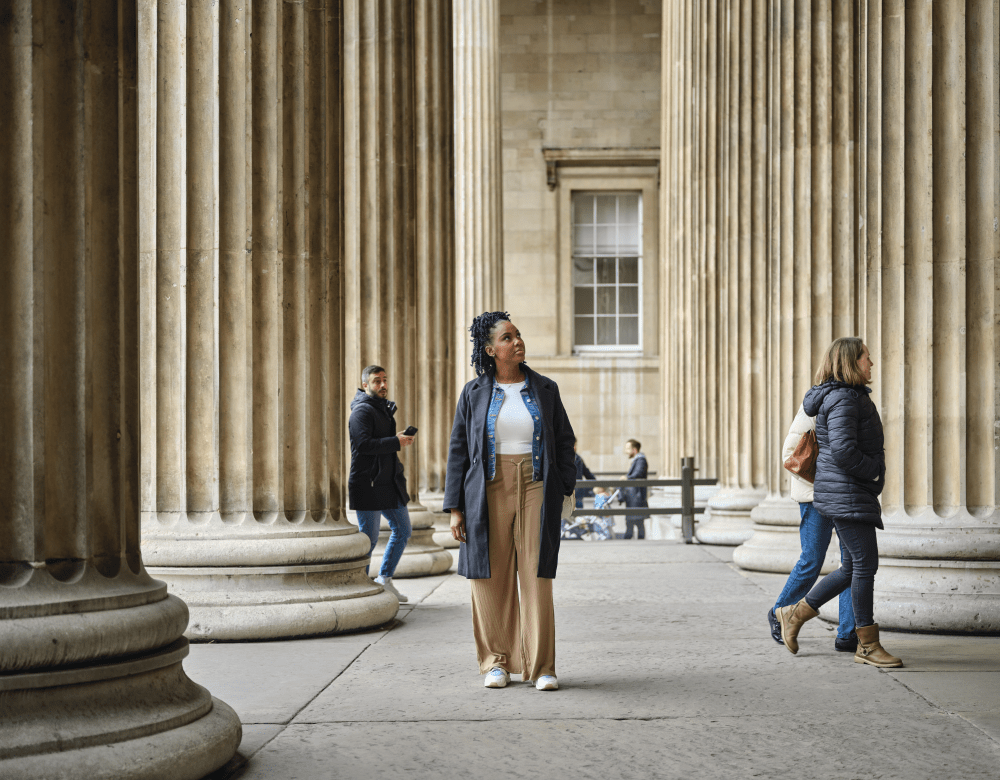
CRISPR-Cas9, instructions for use
Since 2012, successive improvements have made this genome-editing tool more versatile and more precise (not available in English).
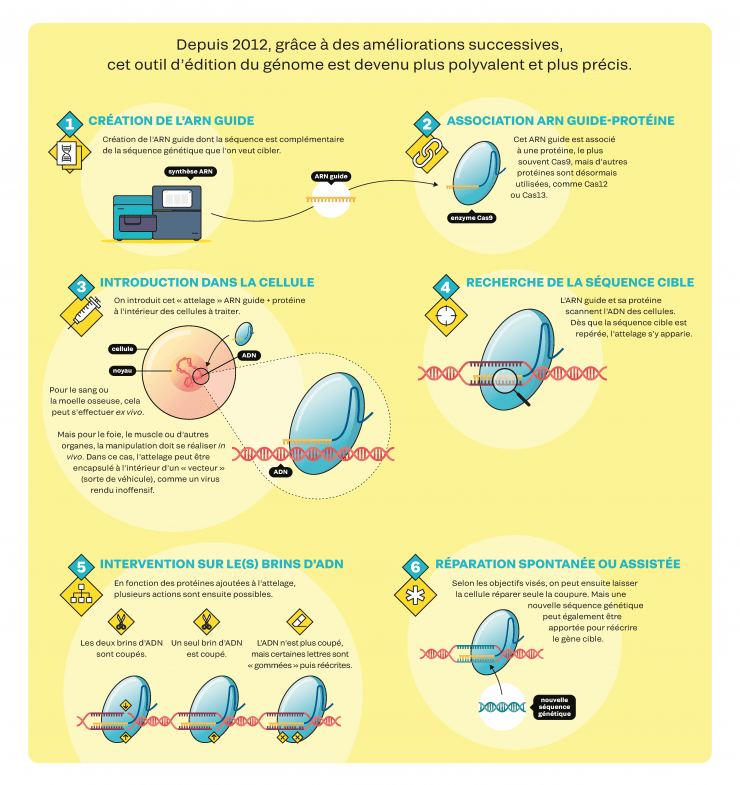
A revolution in the labs
In biology, there is clearly a “before” and an “after” Crispr. Using this toolbox is quick and easy. In addition, there are no patents to restrict its use in basic research: all you need to know is the genetic sequence you are looking for, and you can search the vast online libraries for constructs already developed by other teams and order them for less than 100 euros. This is why most biology laboratories now use Crispr-Cas9 and its derivatives on a daily basis. Many use it to study the role of genes and their mutations: thanks to Crispr, you can easily observe the consequences of deactivating a gene. Others use it to modify human cell lines in vitro, to better understand the development of a disease or to test molecules for therapeutic purposes. Still others modify the embryonic cells of laboratory animals so that, once they are adults, all their cells contain the modified DNA. This makes it possible, for example, to generate new models of human genetic diseases. “It's disruptive technology. Research that used to take more than a year can now be carried out in a few weeks, at a cost that is ten times lower”, points out biochemist Rodolphe Barrangou, director of the CRISPR Lab at the University of North Carolina in the United States. It should be noted that, while the scientific community has free access to the technique, commercial use requires the payment of royalties to patent holders. There are more than 11,000 patents relating to Crispr, depending on the proteins used, their purpose, etc.
Crispr is already on plates
In May 2023, the United States authorised the experimental consumption of pork genetically modified using Crispr. Aimed at improving meat quality and livestock resistance to climate change, this research project involves rendering pigs sterile and then transplanting stem cells from donor animals, capable of producing sperm, into their testicles. In the United States, Canada and Japan, “crispr” foods have been on sale since 2014: herbicide-resistant rapeseed, fish that grow faster, a mushroom that does not oxidise, etc. In France, no “crispr” food has yet been authorised for cultivation or marketing.
To be or not to be a “new GMO”?
Should plants whose genome has been edited using these new genomic techniques be considered GMOs? Yes, said the Court of Justice of the European Union in 2018: the DNA has indeed been modified directly by human hands. But for the industry, as long as no new genes are added, “we are merely accelerating modifications that could occur in nature or by selective cross-breeding”. This is a rather hasty dismissal of the issue of traceability: current techniques do not always make it possible to identify these deliberate DNA modifications. In July 2023, the European Commission will put forward a new legislative proposal. If Parliament approves it, probably not before the European elections in 2024, plants whose DNA modifications could be a result of natural selection will no longer be considered as GMOs. They will be able to be grown and marketed without any particular label (except in organic farming, where they will continue to be banned). In a simple declaration, seed companies will have to prove that the genetic modifications induced can be obtained naturally. On the other hand, if the modification cannot occur spontaneously, or if it is intended to make the plant resistant to plant protection products, it will still require authorisation and will have to be labelled “NGT” (New Genomic Techniques). This change in approach could lead to the development of more abundant crops that are more resistant to climatic conditions. But it also could make farmers even more dependent on a handful of corporations who have begun to patent these seeds on a massive scale.
A tool capable of detecting everything?
Using Crispr to detect genetic segments of viruses (Zika, Covid, dengue, ’flu, etc.), bacteria (tuberculosis, staphylococcus, listeria, etc.) and tumours (specific microRNAs) would considerably improve screening. As no special equipment is required, these new diagnostic tools could be used directly in the field: a major advantage in the event of an epidemic. In 2020, the first Covid self-test based on this technology was authorised in the United States. Most of these tests use proteins other than the famous Cas9: when they detect the target genetic segment, a fluorescent signal is produced, which serves as a visual indicator.
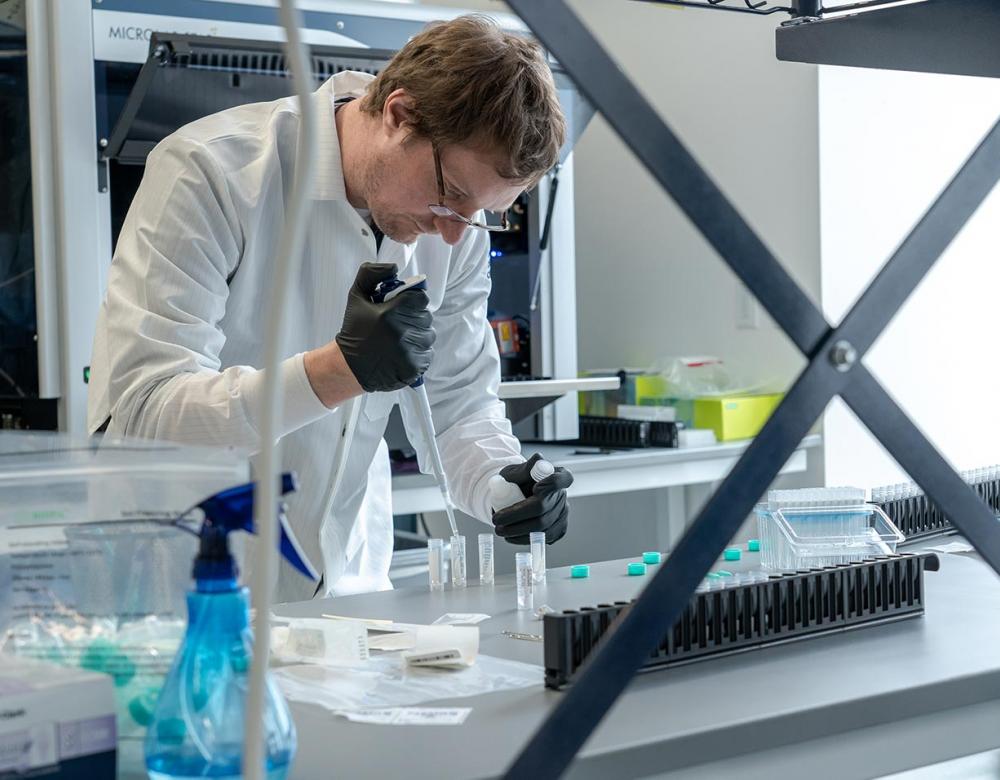
Potential applications in medicine
By correcting a mutation or replacing a mutated one with a healthy one, Crispr represents an immense hope for curing genetic diseases, in particular the 7,000 “monogenic” diseases, which result from a mutation in a single gene. The most advanced clinical trials concern sickle cell anaemia and beta-thalassaemia. In the case of blood diseases, treatment is carried out ex vivo: blood cells are harvested, modified using Crispr, multiplied and then re-injected by transfusion. Other clinical trials are focusing on eye diseases, such as Leber Congenital Amaurosis, because the cells are found on the surface of the body and are therefore easy to access. Still others, on liver diseases such as hereditary transthyretin amyloidosis: scientists have developed vectors to deliver the Crispr system to the liver. However, it is the field of oncology, in particular blood cancers, that has the most ongoing clinical trials. The idea is to genetically modify T-lymphocytes, immune cells, to make them capable of identifying and attacking diseased cells. One to three years after undergoing such treatment, around twenty lymphoma patients are now in complete remission. Finally, the fight against infections could benefit from this tool, which is capable of targeting the genetic sequence of a pathogenic bacterium. A clinical trial to combat AIDS has also begun in the United States. The aim is to generate a mutation that prevents HIV from penetrating immune cells.
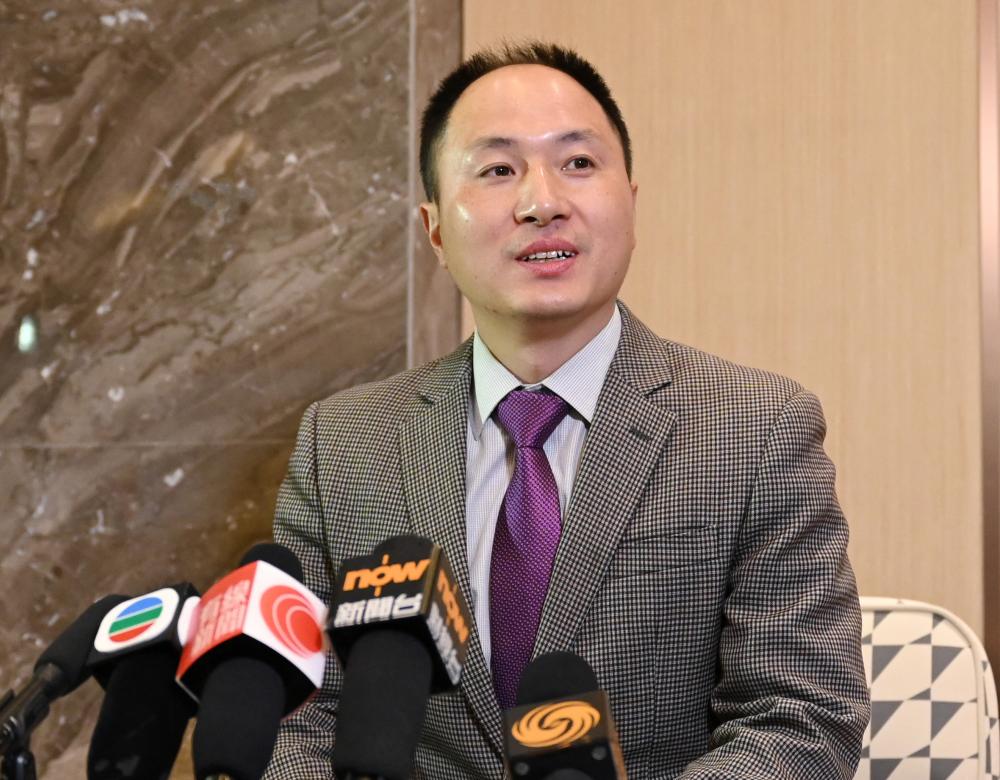
The chilling spectre of “designer babies”
While Crispr can be used to modify adult cells, it can also be used – ethics aside – on embryonic cells! In 2018, the Chinese biologist He Jiankui announced that he had modified human embryos using Crispr-Cas9: three children were born carrying a mutation that confers resistance to AIDS. The scientific community strongly condemned the experiment, given the lack of sufficient guarantees as to its efficacy and safety, and the fact that the conditions for informed consent by couples were not met. The researcher, who spent three years in prison for “illegal medical practice”, wishes to continue his research, but now using adult cells.
Many doubts and challenges remain
Correcting a defective gene in cells in vitro is one thing. Achieving it in vivo, in the right place and without risk, is quite another. One of the main difficulties with the Crispr system is transporting it to the target organ. The most commonly used method is viral transport: viruses rendered harmless carry Crispr and its associated proteins to the nuclei of the target cells. By proliferating, this vector enables Crispr to be produced continuously, and is therefore highly effective. But immune reactions to these viral vectors have led to failure and even death in a clinical trial in the United States. Other vectors are being tested, including the lipid nanoparticles used in vaccines against Covid-19. As for the safety of the tool itself, several risks have been identified. The Crispr system can “cut” in the wrong place, on a genetic sequence resembling the target sequence. It can make correction errors in the target. It can trigger unwanted cellular repair mechanisms, particularly when two DNA strands are cut. Finally, it can generate unexpected interference with other genes. “When millions of cells are affected, as is the case in therapeutic applications, we really need to make sure that these unwanted effects do not occur in the treated cells”, warns biophysicist Carine Giovannangeli (MNHN). For the time being, however, the data available, with monitoring extending to four years, is reassuring. Several marketing authorisations for therapeutic applications are expected over the next two years.
A tool capable of eradicating a species?
An international team of researchers has used Crispr-Cas9 to render female mosquitoes and their offspring sterile. Carried out in 2021 in large cages in Italy, the experiment shows that an entire population can be wiped out in the space of a year. What was the aim? To get rid of these malaria vectors. Full-scale trials are planned in five to ten years’ time. However, this technique known as a “gene drive” is raising concerns: apart from the risk of an exchange of genes and therefore a possible transfer of the Crispr system to other closely-related species, the disappearance of a species has an impact on the rest of the ecosystem that is difficult to predict.
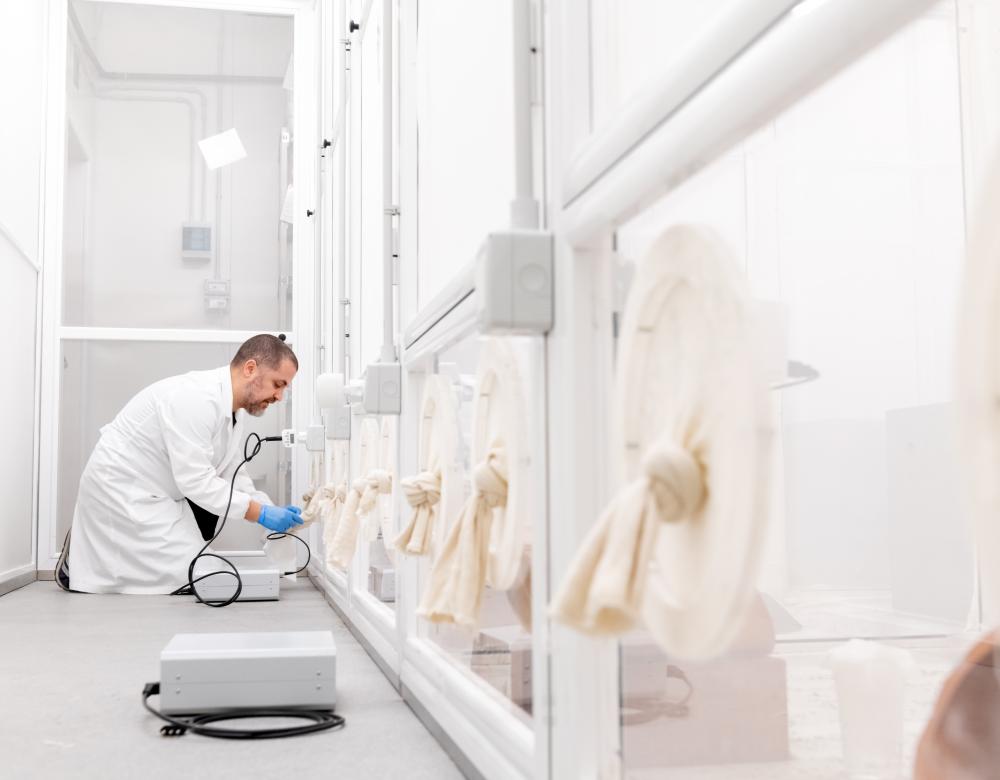
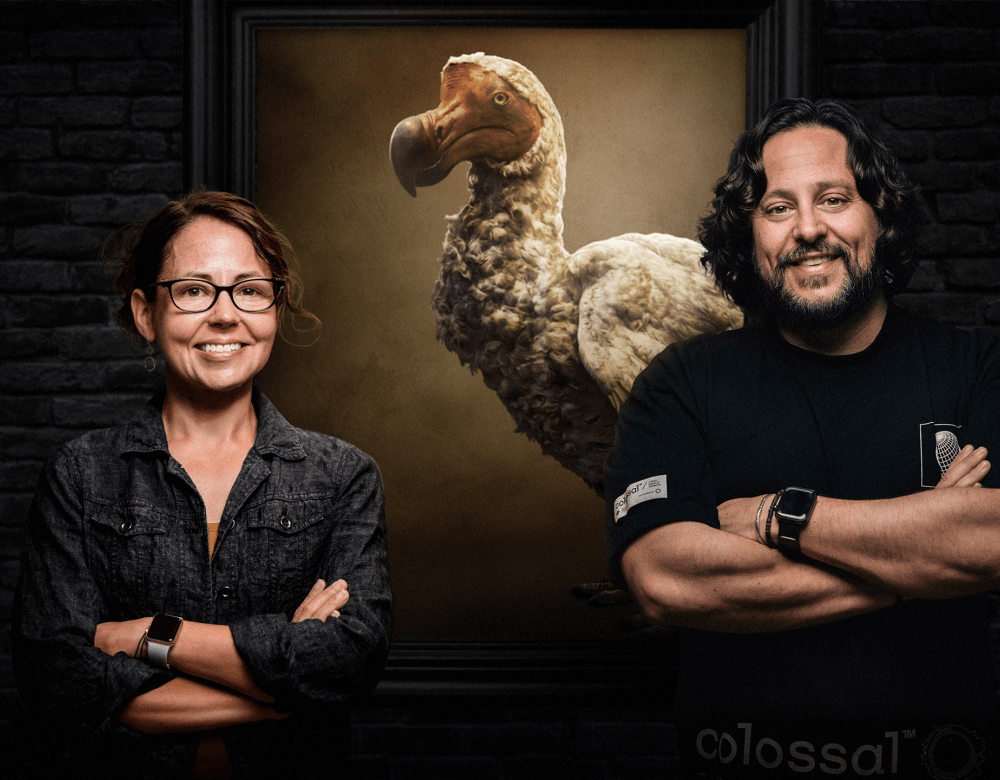
A tool capable of resurrecting a species?
Palaeogeneticist Beth Shapiro, a member of Colossal Biosciences' Scientific Advisory Board, and the company's co-founder, Ben Lamm. By comparing the genome of a dodo, taken from a specimen kept in a museum, with that of the Nicobar pigeon, its closest living relative, American researchers have detected the main genetic differences between the two. Using Crispr, they plan to rewrite the pigeon’s genome to bring it closer to that of the legendary extinct bird. The modified cells would then be inserted into an egg from which a new first dodo could hatch. Other so-called “de- extinction” projects involve the woolly mammoth and the Tasmanian wolf. These are controversial projects, not least because it is unlikely that these animals will survive in the wild today.
A tool capable of saving forests?
Researchers at the University of North Carolina are using Crispr to create new varieties of eucalyptus and poplar that grow faster and are more resistant to natural threats such as drought, frost and disease. Whereas it used to take at least fifteen years to select and produce improved varieties, it now takes just a few months to modify several genes of interest, test their impact and produce the best specimens by cloning.
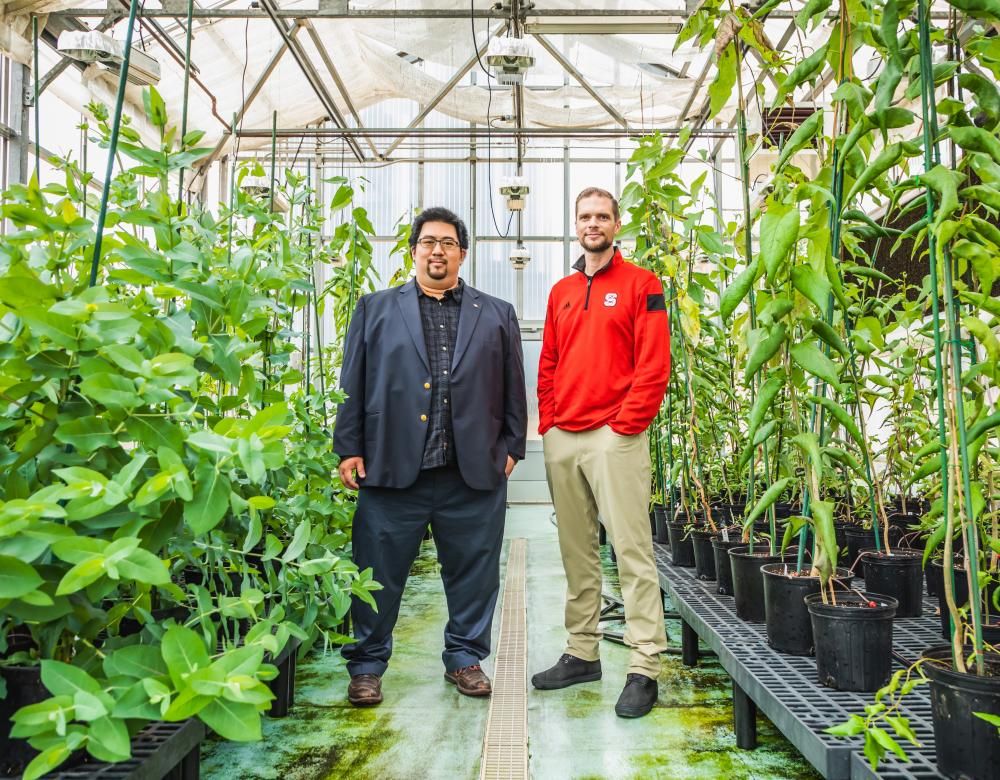
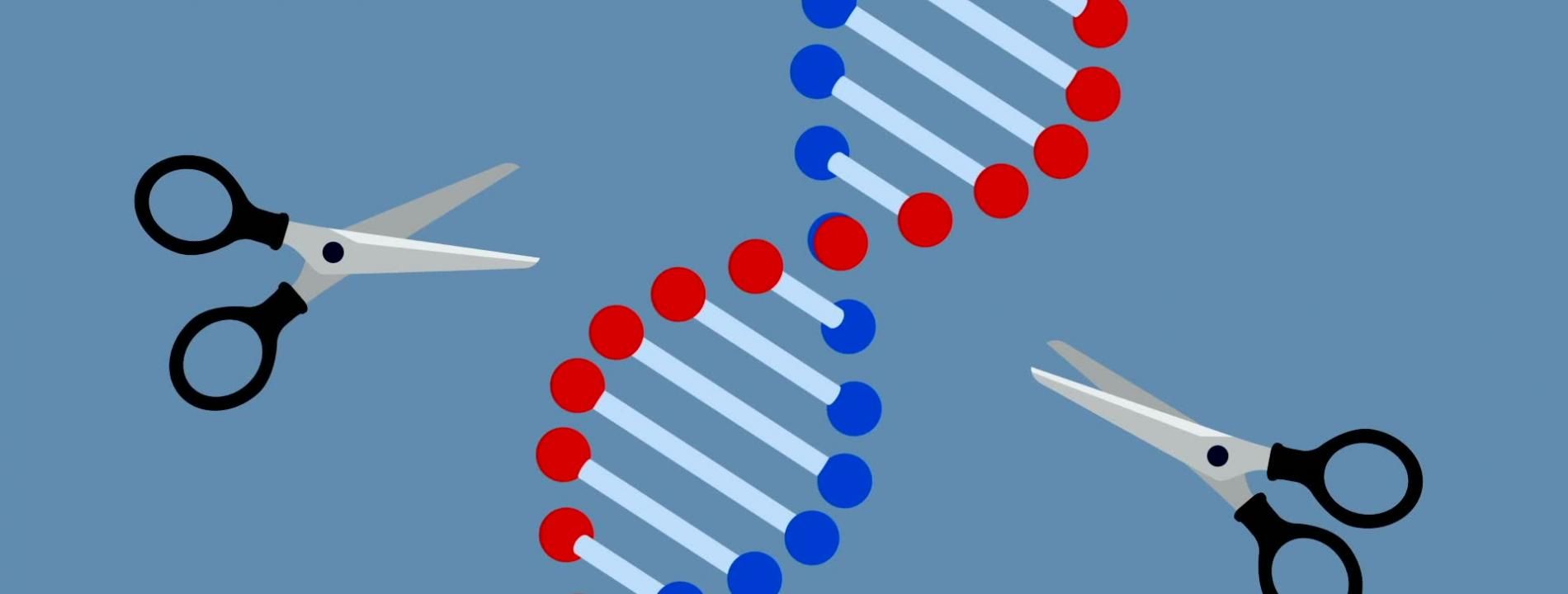